- Polymer and Polymer Agents
- Polymer Enhanced Oil Recovery Mechanisms
- Polymer Property Evaluation Methods
- Polymer Project Data Description
- Polymer EOR Screening Criteria Update
Polymer and Polymer Agents
The word polymer comes from the Greek words polys, meaning many, and meros, meaning parts (Chatterji & Borchardt, 1981). A polymer is a very large molecule made up of thousands of repeating blocks. These blocks, known as monomers, join to form polymers, resulting in long chain molecules. A polymer is a homopolymer if it has one monomer, an adimer if it has two monomers, and a copolymer if it has two different monomers. Copolymers are used commonly in polymer chemistry to represent the joining of two different monomers. Polymer molecules are soluble in water due to the hydrogen bonding between water molecules and the polymer’s polar side chains (Donaldson et al., 1989), which increases the water viscosity. Polymer flooding processes use many molecules of a high molecular weight to alter the flow of water.Polymers often are used in drilling as either fracturing or completion fluids, and in injection well profiles as blocking agents (Chatterji & Borchardt, 1981; Taylor & Nasr-El-Din, 1995). To control mobility, however, the polymers most widely used in oil field recovery projects include hydrolyzed polyacrylamides (HPAM), polysaccharides (e.g., xanthan gum), and hydrophobically associating polymers (AP). A variety of these polymers are available from different manufacturers.
Hydrolyzed Polyacrylamide (HPAM)
Polyacrylamides (PAM) are synthetic polymers available in both powder and liquid (emulsion) form (Needham & Doe, 1987; Chang, 1978; Donaldson et al., 1989). These polymers are manufactured in a variety of ways to yield a wide range of physical and chemical properties that make them candidates for many different oilfield applications. Polyacrylamides are made through the polymerization of acrylamide monomers (Gao,1987; Foshee et al.,1976).Polyacrylamides adsorb onto mineral surfaces of porous media; to reduce polymer adsorption, the macromolecules undergo a process of hydrolyzation. In this process, the polyacrylamide molecules react with a base (sodium hydroxide, sodium carbonate, and potassium hydroxide), which converts some of the amide groups (CONH2) into carboxyl groups (-COO-) (Aluhwal, 2008; Green & Willhite, 1998; Muller et al., 1981; Flory, 1953; Martin & Sherwood, 1975), as shown in Figure p1.
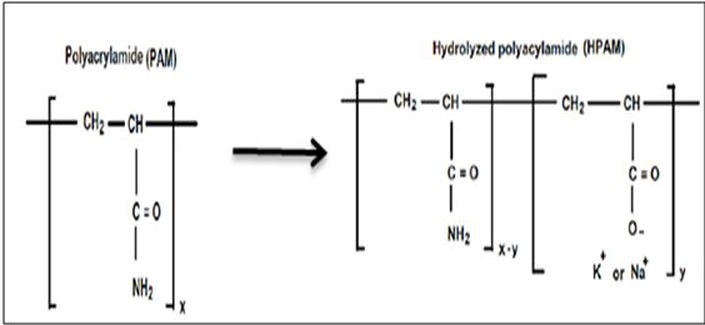
Figure p1. Structure of polyacrylamide and partially hydrolyzed polyacrylamide (Aluhwal, 2008)
Hydrolyzed polyacrylamide adsorption on the rock surface decreases because both the carboxyl groups and the mineral surfaces have a negative charge (Aluhwal, 2008; Green & Willhite, 1998; Hirasaki & Pope, 1974). In addition, partially hydrolyzed polyacrylamides can increase the viscosity of water more than unhydrolyzed polyacrylamide due to the electrical repulsion between the negatively charged carboxylate groups on the polymer chain. This repulsion causes the polymer chains to extend, thus increasing both the fluid mechanical volume of the polymer and the viscosity (Martin & Sherwood, 1975; Yabin et al., 2001). The viscosity of the salting solution decreases because the salt ions weaken the electrostatic resistance among the chains, causing them to coil. This coiling effect first decreases the fluid mechanical volume and then the viscosity of the salting solution. The degree of hydrolysis is measured by the ratio of the number of carboxylate groups to the number of acrylamide and carboxylate groups (Gao, 1987). While it is important to hydrolyze the polymer to prevent adsorption, if the degree of hydrolysis is insufficient, the polymer’s solubility will decrease in water. If the degree of hydrolysis is excessive, the polymer’s viscosity will decrease due to sensitivity to hard water (Green & Willhite, 1998; Ahmad, 2000; Wang et al., 2003; Sheng, 2010). The optimum degree of hydrolysis is between 15% and 33% (Green & Willhite, 1998).
An HPAM with a greater degree of hydrolysis will cause greater viscosity reduction in the presence of divalent ions. This reduction is caused by the interaction of a larger number of anionic and cationic species (Martin & Sherwood, 1975; Ward et al., 1981). The viscosity decreases as the salinity increases, as shown in Figure p2 (Dong et al., 2008).
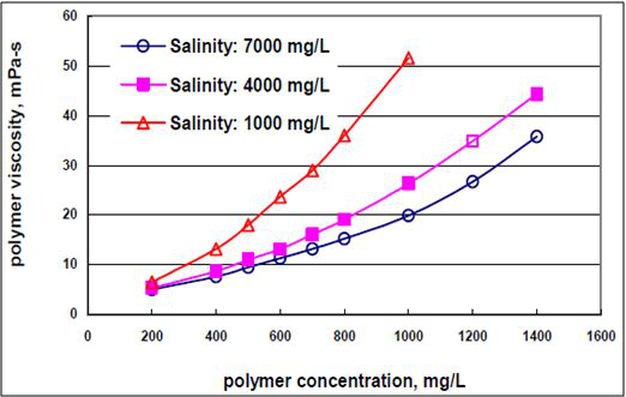
Figure p2.Viscosity of hydrolyzed polyacrylamide in distilled waters (Dong et al., 2008)
Many researchers have studied the effect of both divalent and monovalent ions on the viscosity of HPAM (Mungan, 1972; Ward et al., 1981; Martin & Sherwood, 1975; Jennings et al., 1971). Previous research has proven that divalent cations reduce the solution viscosity of HPAM more so than monovalent cations. Furthermore, calcium ions (Ca 2+) affect viscosity loss more than magnesium ions (Mg2+) (Ward et al., 1981).
Martin and Sherwood (1975) studied the effect of the hydrolysis of a polyacrylamide on the solution’s viscosity. The results indicated that the behavior of polyacrylamide depends on both the nature and extent of the hydrolysis of the polymer molecule. The higher the percent of hydrolysis, the more the polyacrylamide is affected by the divalent ions (Ca2+ and Mg2+), as shown in Figure p3.
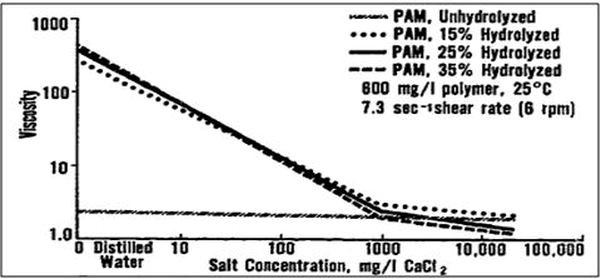
Figure p3. Viscosity of hydrolyzed polyacrylamide in calcium chloride waters (Martin & Sherwood, 1975)
In addition, hydrolyzing polyacrylamide will increase the viscosity in fresh water and sodium chloride (NaCl) brine s, as shown in Figures p4 and p5.
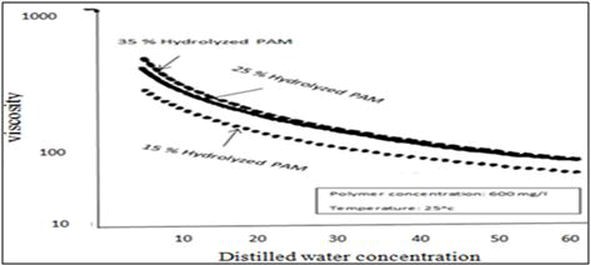
Figure p4. Viscosity of hydrolyzed polyacrylamide in distilled water (Martin & Sherwood, 1975)
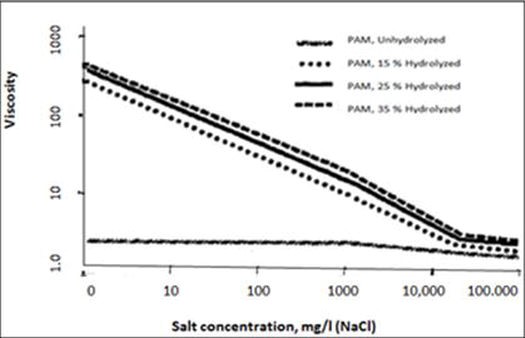
Figure p5.Viscosity of hydrolyzed polyacrylamide in sodium chloride brines (Martin & Sherwood, 1975)
Polysaccharide (e.g., xanthan gum)
Xanthan, a biopolymer used in field recovery projects, is supplied as either a dry powder or a concentrated broth (Green & Willhite, 1998). Polysaccharides are produced from a micro-organism fermentation process that uses the bacterium xanthomonas campestris (Needham & Doe, 1987; Salamone & Clough, 1675). The basic polymer structure is composed of three different saccharide monomers, mannose, glucose, and glucuronic acid (sugar molecules). Side chains, as shown in Figure p6, give the molecule a rigid, helical structure.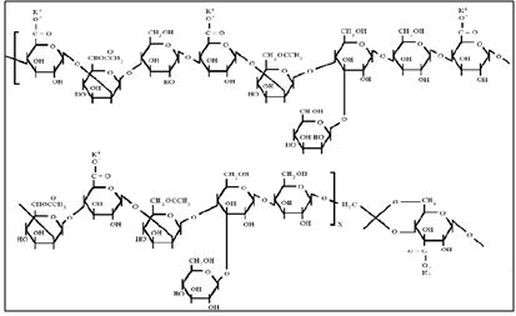
Figure p6. Molecular structure of xanthan gum (Aluhwal, 2008)
These side chains shield and protect the backbone of the polymer molecule from not only enzymatic attacks, but also backbone cleavage. The complex structure makes the xanthan gum less sensitive to both the salt concentration and pH. In addition, this structure allows xanthan to tolerate the mechanical shearing effect. One of the advantages of xanthan gum is that it viscosities in high-salinity water and is resistant to shear degradation (Chang, 1978; Sheng, 2010; Donaldson & Chilingarian, 1989). Its primary disadvantages are formation plugging and susceptibility to bacterial attack (bacterial degradation). However, both problems can be solved by filtration or additional processes. Filtration removes the undesirable cellular debris remaining from the manufacturing process, while adding bactericides can prevent bacterial degradation (Chang, 1978; Unsal et al., 1979; Chauveteau & Kohler, 1984). As a result of these additional processes, xanthan gum costs more than other polymers (Chang, 1978).
Associating polymers (AP)
Associating polymer (AP) is a new kind of polymer being used for polymer flooding. It contains acrylamide monomers and a small percentage of hydrophobic monomers <1% that attach to the polymer’s backbone during the polymerization process, as shown in Figure p7 (Zhou et al. 2007).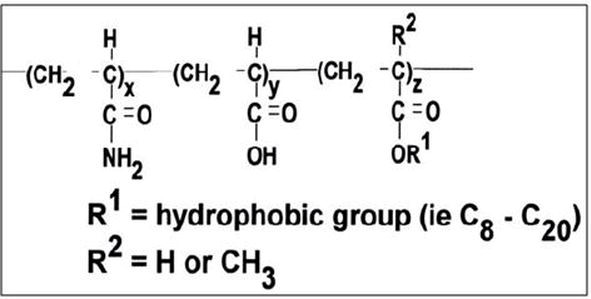
Figure p7. Structure of hydrophobically associating polymers (Zhou et al., 2007)
The hydrophobic groups interact to form an associative polymer in water solution as shown in Figure p8 (Taylor & Nasr-El-Din, 2007).
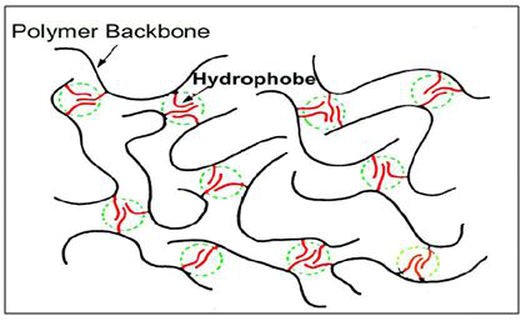
Figure p8. Interaction of hydrophobes in associating polymers (Taylor & Nasr-El-Din, 2007)
That interaction, however, will generate superior viscosities at a similar molecular weight. Moreover, the interaction is unaffected by the addition of salt; as a result, the viscosity of the polymer is not sensitive to salinity, unlike in other polymers (e.g., HPAM). Figures p9 and p10 illustrate how the viscosity of the polymer increases when compared to HPAM as a function of both the polymer and NaCl concentration (Yabin et al., 2001).
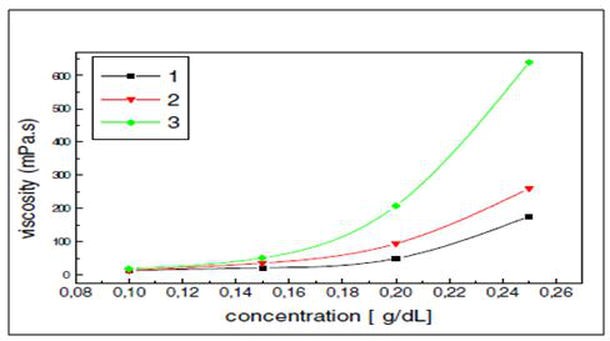
Figure p9. Polymer viscosity of the HPAM (1) and AP (2 and 3) as a function of concentration at 45oC (Yabin et al., 2001)
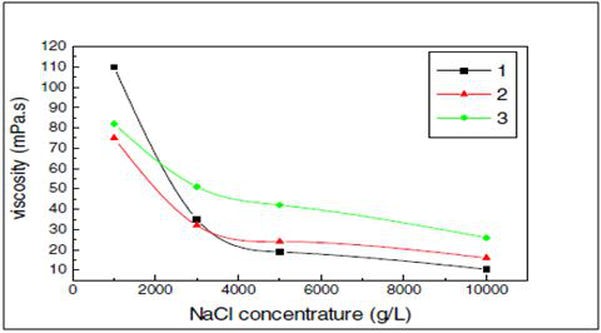
Figure p10. Polymer viscosity of the HPAM (1) and AP (2 and 3) as a function of NaCl concentration (Yabin et al., 2001)
Studies have reported that oil recovery increased 6% more when using the AP instead of the HPAM solution in core flood experiments (Yabin et al., 2001; Reichenbach et al., 2011).
Go To Main Menu |
Continue To Polymer EOR Mechanisms |